Physicists Explore New Methods of Searching for Dark Matter
August 10, 2022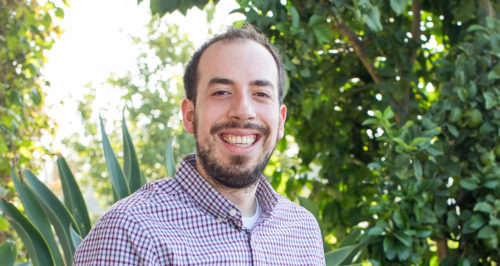
Experimental searches in Harvey Mudd College physics professor Brian Shuve’s field of dark matter and particle physics could be compared to searching for a needle in a haystack—if the needle is invisible. A leader in the field of dark matter physics, Shuve has been proposing new ideas to look for viable theories of dark matter in collider experiments, and has also been analyzing data from lower-energy particle collision experiments that could offer new insight into dark matter. Physical Review Letters and Journal of High Energy Physics have published research papers on the topic by Shuve and his students.
Two of the papers are based on analysis the Shuve group did of data from the BABAR particle physics experiment that ran from 1999 through 2008 at the SLAC National Accelerator Laboratory. The Shuve group took the approach of sifting through existing datasets to look for signals of dark particles. “We did searches for two types of dark forces that are common in dark matter models,” Shuve says. “I’ve been motivated by the fact that these one or two baseline scenarios are probably overly simplistic and, while they serve as good benchmarks, there is a lot more that these experiments can do that can be missed with current approaches.”
Physical Review Letters published “Search for a Dark Leptophilic Scalar in e+e− Collisions” in 2020 and has released the preprint of “Search for an Axion-Like Particle in B Meson Decays.” Ina Flood ’20 and Ngan Steve Nguyen PZ ’21 are co-authors on the latter paper. “These were analyses with major HMC contributions,” says Shuve. “It is extremely rare for a primarily undergraduate institution to do publishable research with particle physics experiments, so I am especially excited about this work.”
So, how does one detect dark matter? “Because of E = mc2,” Shuve says, “it is possible to take the energy in the particle collision and turn it into new kinds of mass, including dark matter. There is then the question of how we detect dark matter if it is made. If it is truly dark matter, then it is invisible and just escapes from the collision. The way we find it is by looking for something missing.”
Shuve explains further, “In physics, there are deep principles called conservation of energy and conservation of momentum that relate energy and the motion of particles before and after the collision. If some of the energy and momentum escapes in the form of dark matter, if we add up all the pieces before and after the collision, then we can see that the sums don’t check out. If there are multiple kinds of dark matter particles, then it is possible that a heavier dark matter particle will decay into the lighter one, releasing energy in a form we can detect (like light). So, we have to consider lots of possibilities to make sure we don’t miss it!
“Ultimately, we are always measuring energy from the collision—what is the energy of the particles going into the collision, what is the energy of the particles coming out of the collision and what might be missing? We are also keeping track of the types of particles coming out of the collision and how the energy is distributed among them,” Shuve says. “To look for rare processes, we are looking for particular combinations of particles and the distribution of energies among these particles that is uncommon in our current theory of particle physics but might be a common prediction of a dark matter theory. Because these experiments collect so much data (typically millions of collisions a second), there is a needle in a haystack problem; we need to have an idea of what we are looking for in order to dig it out.”
In addition to this experimental work, Shuve, Mason Acevedo ’22, Albany Blackburn ’23 and Mavis Stone ’23 conducted a theoretical simulation-based study of new ways that experimentalists can look for evidence of dark particles at BABAR and a new experiment called Belle II. Last year, the Journal of High Energy Physics published the results, “Multi-track displaced vertices at B-factories.”
“I’m always looking for new and creative things we can do with these experiments,” Shuve says. “I’ve spent a lot of the last seven or so years rolling up my sleeves and getting involved with the experimental analysis in addition to theory proposals. This has allowed me to get actual results rather than just trying to convince experimentalists to do the work and has given me insight into the opportunities and challenges of doing experimental searches, as well as allowing me to make realistic estimates for what the experiments can achieve.”
Shuve attributes his creativity in thinking about new experimentation to the time he has spent researching dark matter and hidden sector models at higher energy colliders, especially the Large Hadron Collider at CERN. “Because the high- and low-energy colliders are built with different purposes in mind, the approach of experimentalists is usually different between the two communities. By having interests that span both, I’m able to bring expertise and experience gained at the LHC and bring that mindset to think of new ways to use these lower energy experiments,” he says.
Unfortunately, Shuve says, he and his students did not find evidence of new particles in existing datasets. However, he says, “We still learn something even if we don’t detect new particles: the fact that we didn’t see something allows us to set limits or constraints on the possible properties of these particles. In other words, if they have properties that should have shown up in our data and we didn’t see them, then that theory is ruled out. We can then take these constraints and compare them to the predictions of dark matter theories based on astrophysics or cosmology, and so we can tell whether we’ve completely ruled out a particular theory or if we need to modify the experiment in some way to test it better.
“For the particular searches we’ve done, we have improved constraints by up to a factor of 100 or more on the interaction strength of the new particles. This is a huge improvement—based on pre-existing constraints, it’s possible we could have seen 10,000 or 100,000 collisions with evidence for the new particles, so when my students and I finally looked at the dataset, we could have truly discovered something spectacular! We didn’t, and now a range of parameters in the theory is ruled out.”
And, after all, just because they haven’t seen evidence of new particles yet, doesn’t mean they’re not there.